Recently, a group of physicists from the Institute of Modern Physics of the Chinese Academy of Sciences has manufactured for the first time an antimatter hypernucleus, the heaviest observed to date: antihyperhydrogen-4. As it could not be otherwise, the work has been published in Nature.
But what is this antimatter? Is it as dangerous as Dan Brown made us think in his best seller Angels and Demons? Well yes, but no. The reality is that it is so difficult to manufacture that it would take us billions of years to accumulate a worrying amount.
Antimatter, the archenemy of matter
In a way, matter and antimatter are public enemies, because if they met they would annihilate each other without hesitation, but let’s be more technical.
Antimatter is made up of antiparticles. An antiparticle has the same mass and the same state of spin than its corresponding particle of matter, but with an inverse charge (let us ignore another series of quantum properties here).
An example of a particle-antiparticle pair is the electron and the positron. The fundamental difference between the two is their electrical charge. That of the electron is negative and that of the positron is positive. The rest of the properties, such as mass and spin, are the same. Its annihilation can give rise to photons of different energies and other heavy particles, depending on the initial kinetic energy they had before being destroyed.
Standard antiparticle model
It has been just over a century since the first subatomic particle was discovered. It was in 1897 when J. J. Thomson announced the corpuscular nature of the cathode rays and the discovery of a particle he called a corpuscle. Later it took the name electron.
After this milestone, a large number of fundamental particles have been discovered. Bosons, responsible for fundamental interactions or forces, and fermions, which make up matter. Therefore, for each boson there must exist an antiboson.
The first antiparticle detected was the positron, in 1932, by Carl Andersonwhile studying cosmic rays using a cloud chamber. About 20 years later, Segré and Charberlain used a particle accelerator to determine the existence of the antiproton and just one year later (1956) and in the same laboratoryBruce Cork and his team were able to detect the antineutron. The last elementary antiparticle detected was the quark antitop (anti-above), in 1995.
Let’s create antimatter!
“Hold my tank…!” was probably what Professor Walter Oelert told his colleagues at the European Laboratory for Elementary Particle Physics (CERN) back in 1995. His group was the first to create antimatter atoms.
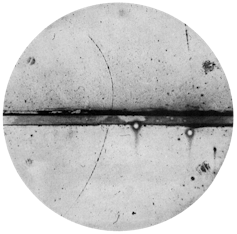
The process was complex, since it involved creating antiprotons and positrons (antielectrons), separately, and then joining them to form the antiatom. Of course, the half-life of these antiatoms is about forty billionths of a second (what it takes for light to travel 10 meters in a vacuum), immediately annihilating themselves with the surrounding matter. Keeping it isolated to study it seemed like an impossible task.
But you don’t need to have a particle accelerator, like CERN, in your backyard to create antimatter; it also occurs naturally in some radioactive decay processes of atomic particles or nuclei. An example of these is the beta decay positive of the proton within an atomic nucleus, producing a neutron, a positron and an electron neutrino. These decays can and are used for cutting-edge applications such as radiodiagnostics.
positron emission tomography
Human beings use antiparticles. The positron emission tomography (PET) is a medical technique widely used in the diagnosis of tumors, neurodegenerative diseases and cardiac conditions. PET is based on the annihilation of positron-electron pairs. The process generates gamma rays that are collected by the diagnostic equipment.
Positrons are generated by the decay of a radiopharmaceutical, normally Fluorine-18, and, based on the information captured by the detectors, a three-dimensional image is reconstructed of the areas where the positron emission occurred. This indicates the areas of the body where the radiopharmaceutical accumulates in greater quantities, which reflects the metabolic activity of those tissues.
Symmetry is broken
How we physicists like symmetries… And everything, or almost everythingit is nicer if it is symmetrical. But with the amount of matter and antimatter in the universe we have not been lucky.
At the moment when the first particles began to appear in the universe, there were the same number of particles as antiparticles, but what happened after? And by after I mean less than a thousandth of a second after the Big Bang. What happened was that the symmetry was broken in favor of matter.
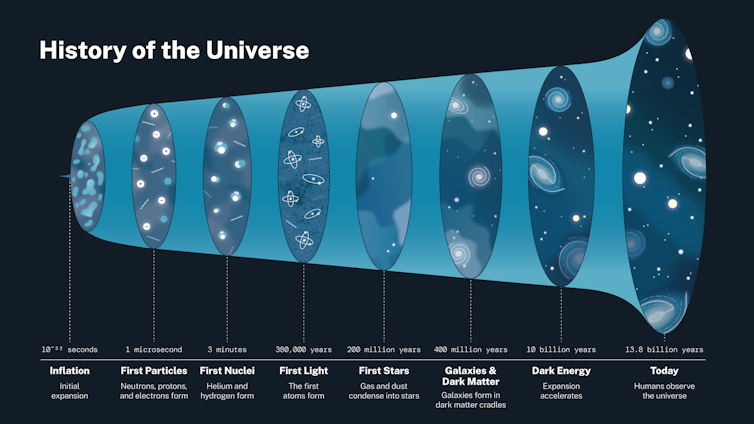
Although there are some candidate theories, the reason is not known for sure, but the amount of matter and antimatter in our universe was fixed at that moment. If there had been other places in the universe made of antimatter, its annihilation with matter would have left a gamma ray signature that would be easily detectable, and that’s not the case, so we don’t know where all that primordial antimatter went.
This is not dark matter
As Alejandro Sanz sings, “it’s not the same…”. Dark matter is not the same as antimatter. Antimatter is visible, tangible, directly measurable. The dark matterOn the contrary, we can only “observe” it through the effect it has on ordinary matter. It is fundamental to explain the rotation of galaxies and also plays a relevant role in the formation of structures at the cosmic level. It was proposed by the astronomer and physicist Fritz Zwicky in 1933 and it seems to correspond to 85% of all the matter in the universe.
Antihyperhydrogen-4
The antihyperhydrogen-4 that physicists at the Institute of Modern Physics of the Chinese Academy of Sciences have created would be the “bad” relative of hyperhydrogen-4, although this still doesn’t mean anything to many of us, right?
The antihypernucleus in question is composed of a hiperón anti-Lambdaone antiproton and two antineutrons.
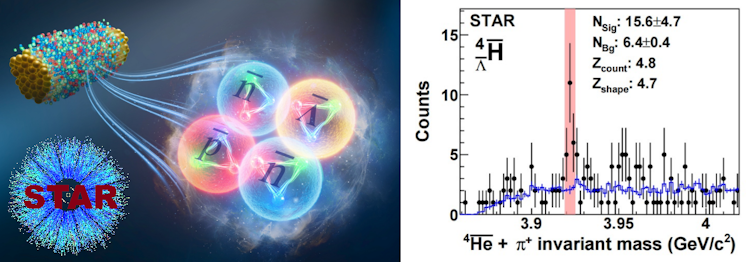
These two nuclei are highly unstable, they quickly decay to lighter particles emitting photons, so the time we can use to study them is practically zero.
Despite this, the team of researchers from the STAR collaboration He used his experiments to study whether or not there was asymmetry between the decay times of the hypernucleus and its corresponding antihypernucleus. They did not observe anything relevant that could support a possible cause of the antisymmetry between the amount of matter and antimatter existing in the current universe.
The questions that arise as we advance our knowledge motivate us to continue researching, developing more advanced technologies and perfecting our theories. This relentless learning process drives innovations that improve life on Earth and bring us one step closer to understanding the complexity of the entire universe.
Francisco José Torcal MillaFull Professor. Department of Applied Physics. Center: EINA. Institute: I3A, University of Zaragoza.
This article was originally published in The Conversation. Read the original.
Source: www.vozpopuli.com